The Noble Gases
The sources detail the history, discovery, properties, abundance, isotopes, compounds, and uses of the noble gases, emphasizing their surprising inertness.
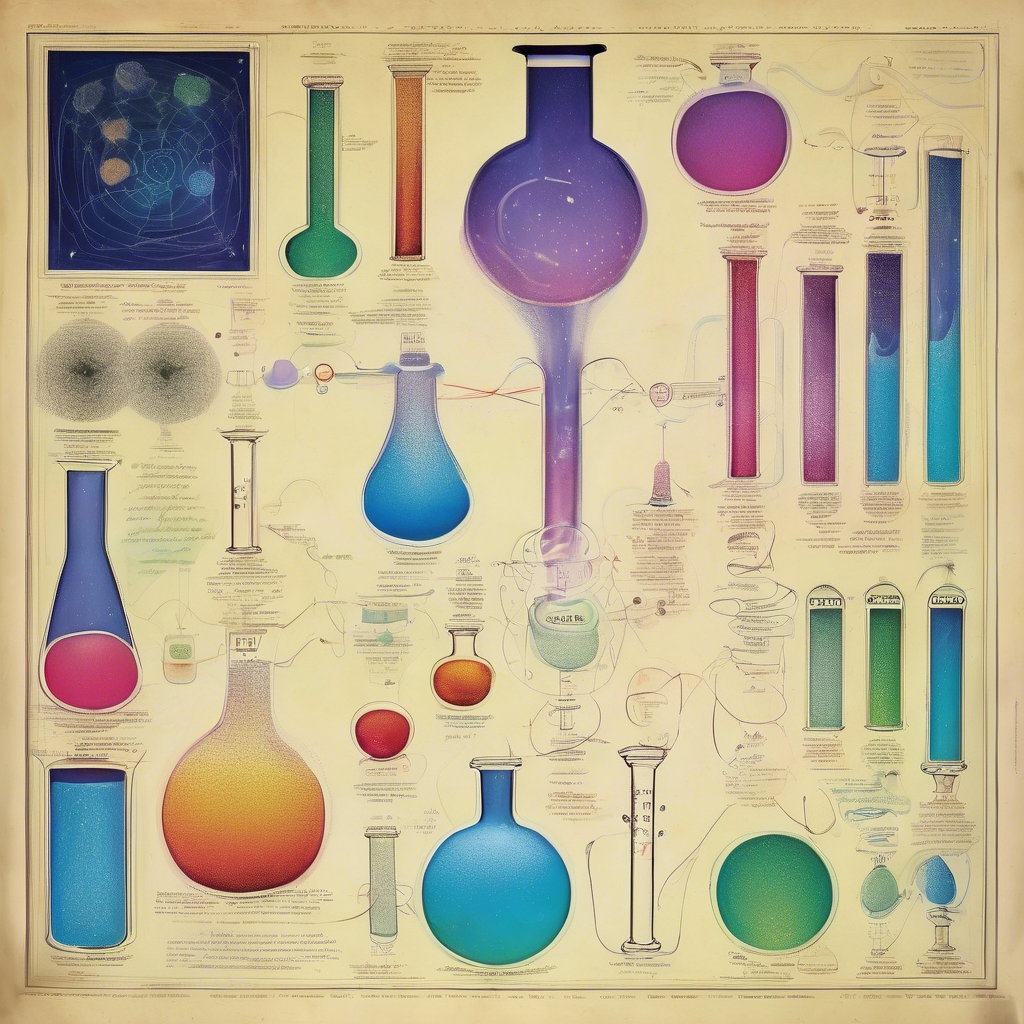
Our story begins in the fading years of the eighteenth century, when the air we breathe was considered a fundamental element, a single, indivisible building block of the universe. Yet, scientists, like Stephen Hales, Joseph Priestley, and Joseph Black, were beginning to devise clever experiments to study gases, separating them and describing their properties. Through painstaking work, the concept of air as a simple element began to crumble. Daniel Rutherford, studying what happened to air when substances burned in it, identified a gas that could not support combustion and in which mice died. Joseph Priestley, heating a combination of mercury and air, discovered a gas in which objects burned furiously. Antoine Laurent Lavoisier later clarified this situation, showing that filtered, dry air was primarily a mixture, composed largely of these two distinct gases: one that supported life and combustion (later called oxygen) and one that did not (later called nitrogen, from Greek words meaning "no life" or "producing niter"). It seemed the composition of air was settled, consisting of about one-fifth oxygen and four-fifths nitrogen, with only tiny amounts of other gases like carbon dioxide and water vapor.
However, the story took an unexpected turn with the work of an English scientist named Cavendish in the late 1700s. He devised an ingenious method using electric sparks to force the two main components of air, nitrogen and oxygen, into combination with each other, forming substances that could be removed by dissolving them in water. His aim was to demonstrate that the accepted proportions of nitrogen and oxygen in air were correct. But in repeating this experiment, Cavendish discovered that after removing all the nitrogen and oxygen he could, a small bubble of gas remained. This leftover gas was unlike nitrogen; it was even more reluctant to combine with oxygen under the influence of electric sparks. Nitrogen, while considered "inert" due to its refusal to combine except under extreme conditions, was positively reactive compared to this residual gas. This strange, seemingly completely inert gas, making up about 1/120 of the original air sample supposed to be nitrogen, was a puzzle that no one followed up for over a century, effectively slipping out of chemical consciousness.
The narrative of this unusual gas surfaced again through a completely different avenue: the study of light from the sun. Pioneers like Isaac Newton had shown how sunlight could be spread into a spectrum of colors. Later scientists like Joseph von Fraunhofer, Gustav Kirchhoff, and Robert Bunsen developed spectroscopic analysis, studying the unique lines that appeared in the spectra of light emitted or absorbed by different substances, allowing them to identify elements based on their spectral fingerprints. In 1868, during a total solar eclipse observed in India, an astronomer, Pierre Janssen, studied the sun's outer atmosphere using a spectroscope and noted a bright line in the spectrum that did not correspond to any known element. Joseph Norman Lockyer, an English astronomer, also studying solar spectroscopy, concluded this line indicated the presence of a new element on the sun, which he tentatively named. This observation, marking the second detection of one of these unusual gases, was also largely forgotten for a generation.
A third instance occurred with an American mineralogist, William Hillebrand, who in 1890, while treating a uranium-rich mineral with acid, collected a gas he believed to be nitrogen. While his spectroscopic analysis confirmed nitrogen lines, he also noted, but did not identify, other lines. In deciding not to pursue these unexplained lines, Hillebrand missed a significant opportunity, having a sample of one of these inert gases in his hands.
The stage was set for the puzzle to finally make itself felt. Lord Rayleigh, an English physicist, was meticulously measuring the densities of gases, partly in an effort to revisit an old hypothesis by William Prout suggesting that all elements might be built from hydrogen. While Prout's hypothesis seemed increasingly disproven by accurate atomic weight measurements, Rayleigh's careful work yielded an unexpected anomaly: nitrogen prepared from the atmosphere was consistently denser than nitrogen prepared from chemical compounds. The difference was small, less than half a percent, but far too large to ignore given Rayleigh's precision. He systematically eliminated possible impurities, like oxygen or hydrogen, or different molecular forms of nitrogen. The evidence pointed stubbornly to some unidentified, denser substance present only in the atmospheric nitrogen.
Rayleigh published his findings, asking for suggestions. This attracted the attention of William Ramsay, a Scottish chemist, who obtained Rayleigh's permission to investigate. Ramsay reasoned that if a denser impurity was present in atmospheric nitrogen, it must differ chemically. By reacting atmospheric nitrogen with red-hot magnesium, which combines with nitrogen to form magnesium nitride, Ramsay sought to separate this impurity based on its reactivity. The process left behind a small volume of gas that was significantly denser than nitrogen and remarkably unreactive, even more so than nitrogen itself. This reminded Ramsay of Cavendish's forgotten experiment. He repeated Cavendish's method using electric sparks and air, also obtaining a small, unreactive bubble. Spectroscopic analysis of this residual gas confirmed it was a new element, showing lines unlike any known substance. In 1894, Rayleigh and Ramsay announced their discovery of a new element. Its extreme inertness led them to name it after a Greek word meaning "inert". Its density explained Rayleigh's puzzle: nitrogen from the air contained this new, denser element, while nitrogen from compounds did not.
The timing of this discovery was crucial. By 1894, the periodic table, organized by Dmitri Mendeleev based on atomic weights and recurring properties like valence, provided a powerful framework for understanding the elements. Placing the newly discovered element in the table presented a challenge; based on its atomic weight determined from its density and monatomic nature, it seemed to violate the expected atomic weight order relative to neighboring elements. However, its complete lack of chemical reactivity suggested a valence of zero, proposing the need for an entirely new column in the periodic table for elements with this characteristic. This implied that if the periodic table was truly valuable, the newly found element could not be unique; a whole family of such gases should exist.
Driven by this prediction, Ramsay, and later his assistant Morris Travers, embarked on a search for other members of this predicted family. Ramsay was alerted by Hillebrand's earlier report of unexplained spectral lines from a uranium mineral. Investigating a similar mineral, he obtained a gas whose spectrum matched the mysterious line seen in the sun's spectrum decades earlier. This confirmed that the element Lockyer had seen on the sun also existed on Earth. Its properties indicated it was another of these inert gases, with an atomic weight fitting the first slot in the predicted zero-valence column.
The search then turned to the atmosphere, the most likely place to find these unreactive gases in free form. While the first found was present in significant quantities (about 1% of the atmosphere), others were expected in much smaller amounts. A new development aided this search: methods for producing liquid air in quantity. By carefully boiling liquid air, a process called fractional distillation, components with different boiling points could be separated. Applying this technique, Ramsay and Travers isolated fractions containing gases with different spectral lines and densities, revealing the existence of other, less abundant members of this inert family. By the turn of the century, a solid group of these gases had been identified, fitting logically into a new column in the periodic table. Their discoveries, driven by the resolution of a density puzzle and the predictive power of the periodic table, earned Nobel Prizes for both Ramsay and Rayleigh.
Further scientific advancements, particularly in understanding atomic structure, including the nucleus (protons, neutrons) and electrons, and the discovery of isotopes (varieties of an element with different atomic weights but the same chemical properties), provided deeper insights into why these elements were so unreactive. Their electron arrangements seemed particularly stable. The study of radioactivity revealed how some of these gases are formed on Earth, like one common isotope of the first found element resulting from the breakdown of a specific potassium isotope, or how some isotopes of heavier members are products of uranium fission. While some members are among the most abundant elements in the universe, others are quite rare, especially on Earth, though some are concentrated in natural gas wells. The lightest member of the family, despite being abundant in the universe, is scarce in Earth's atmosphere because it is so light it leaks into space.
Despite their general inertness, these gases found numerous practical applications. Their reluctance to react made them ideal for protecting hot metal filaments in light bulbs from combining with oxygen. They glowed brightly when electricity was passed through them, leading to signal lamps and advertising signs. Their densities proved useful; the lightness of the second element discovered made it invaluable for lifting balloons and dirigibles as a safe alternative to flammable hydrogen, and its properties made it useful in artificial breathing mixtures for deep-sea divers, reducing the risk of decompression sickness. Other applications arose from their properties at extremely low temperatures, ushering in the science of cryogenics and revealing phenomena like superfluidity and superconductivity. These low-temperature effects led to novel technologies like powerful magnets and tiny electrical switches. Heavier members of the family found niche uses in lighting, detecting leaks, and absorbing X-rays for medical purposes.
However, the greatest surprise was yet to come. For decades, chemists confidently stated that these gases formed no chemical compounds, a belief strongly supported by their properties and position in the periodic table. Despite theoretical suggestions that compounds might be possible under specific conditions, particularly with highly reactive elements like fluorine, early experimental attempts were unsuccessful, partly due to the difficulty and danger of working with reactive substances and the rarity of some of the gases. A practical need during World War II for handling fluorine safely in the separation of uranium isotopes inadvertently developed the necessary techniques. This, combined with renewed theoretical interest, led to new experiments. In a series of groundbreaking experiments in the early 1960s, scientists succeeded in reacting one of the heavier inert gases with fluorine under controlled conditions, forming stable, crystalline compounds. This achievement sparked intense activity, leading to the synthesis of numerous other compounds of these elements.
The discovery that these supposedly inert gases could form compounds was not a shattering blow to chemical theory, but rather a refinement and a powerful lesson. It underscored the importance of challenging assumptions and highlighted that sometimes, scientific progress awaits the development of the right techniques or the removal of practical handicaps. This unexpected turn of events, like so many throughout their history, reminded scientists that certainty can be premature and the universe holds wonders waiting to be uncovered by diligent investigation.
The story of these gases is a testament to the serpentine path of scientific progress, marked by overlooked clues, puzzling observations, persistent investigation, theoretical frameworks, technological advancements, and serendipitous turns.